The objective of the TUM1 subproject is, to develop and understand the Coda technique as a tool for assessing the structural integrity and durability of RC structures. Using well-controllable one-dimensional experiments, this subproject aims to determine the effect of mechanical load and environmental impacts on the Coda signal. On the one hand, calibration curves for the perturbation of the Coda caused by direct impacts will be established. On the other hand, typical concrete phenomena, e.g. the long-term processes carbonation or frost attack, will be characterized in the time domain.
Project description
The subproject TUM1 aims at the experimental and data-based characterization of relationships between external loads and changes in the coda signal. The goal is to provide a spectrum of calibration curves and concrete-specific parameters by the end of the project to enable calibration and signal compensation of real engineering structures. To validate the causes of signal variations, changes in concrete microstructure will be characterized using conventional and innovative techniques. The relationships between impacts and microstructural changes, as well as the corresponding analysis and interpretation of the coda signals, provide important input parameters for modelling wave propagation, material properties, and structural changes, as developed in other individual projects of this research group.
In the second phase, the relationships derived in the first phase through one-dimensional experiments will be systematically extended to include additional influences, such as the surface condition. Furthermore, following the characterization of homogeneous states, moisture and temperature gradients relevant to practice will now be investigated. The second funding phase will also focus on concrete-specific long-term phenomena such as carbonation and frost attack. Special attention will be paid to the distinction between reversible and irreversible microstructural changes. The investigated material changes will be specified through selected methods, such as computed tomography or thin-section microscopy.
Furthermore, the subproject TUM1 is responsible for the coordination of meetings and the public appearance of the research group, as well as the coordination concerning the instrumentation of a real engineering structure and the data acquisition infrastructure for the common database.
Previous Results
In the first funding period, the influence of uniaxial compressive and tensile loads as well as temperature and moisture variations on the coda signal was investigated in controlled laboratory experiments on concrete specimens.
Mechanical Loads
Through uniaxial compression and tension tests, as well as a six-month shrinkage and creep test, it was shown that both the signal correlation of the ultrasonic signals and their velocity variations directly reflect the stress changes in concrete structures. For the elastic range up to 30% of maximum strength, linear regression was used to quantify the relationships between increases in load and changes in velocity variation. The long-term shrinkage and creep test further emphasized that time-dependent material changes must be taken into account when comparing ultrasonic signals that are far apart in time.
Temperature and Moisture
For the investigation regarding the impact of temperature and moisture changes on the ultrasonic signals, concrete specimens with embedded ultrasonic sensors, as well as accompanying specimens with sensors for the determination of the temperature and humidity conditions were produced. The specimens were conditioned in a climatic chamber over several months to moisture conditions of 95% to 35% relative humidity. When a homogeneous moisture distribution was reached, the specimens were subjected to a stepwise temperature profile from 2 °C to 55 °C. Ultrasonic signals were recorded regularly throughout the experiment. The evaluation of the homogeneous temperature and moisture states confirmed previous studies, which reported a linear relationship between temperature changes and seismic velocity variations. Additionally, the relationship between a temperature increase and a change in velocity variation could be quantified for the investigated concrete mixture. Furthermore, an anti-correlation with relative humidity was observed.
Further Investigations
In addition, TUM1 provided material parameters for the enhancement and validation of the material model developed by RUB1. For this purpose, the raw materials as well as the respective concrete were characterized. The characterization included standardized methods such as mercury intrusion porosimetry, compressive strength tests, and the determination of grading curves for the aggregates used. Moreover, tailored experiments were carried out to derive stress-strain curves for the aggregate type used, as well as for concrete and mortar mixes. Further, the usage of neural networks for the accelerated counting of air voids was suggested.
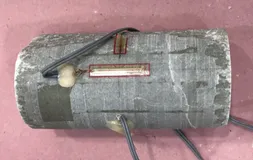
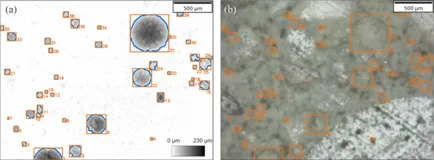